Survival Strategies
It’s not easy being a bug.
There are a lot of “windshields” on the road of life!!
Just try to imagine what it would be like if you were an insect and had to:
- survive rainstorms, windstorms, ice storms, and hail storms,
- find water in the desiccating heat of mid-summer,
- keep from freezing in the dead of winter,
- avoid flash floods, wildfires, and mud slides,
- locate a suitable food supply (host plant or animal),
- elude birds, spiders, mantids, frogs, and other predators,
- defend yourself against parasitoid flies and wasps,
- prevent infection by pathogenic fungi and microorganisms,
- and escape being impaled on a pin in a student’s bug collection!
Despite their small size and apparent vulnerability, insects are not entirely at the mercy of the elements. They are equipped with high reproductive rates and numerous behavioral and physiological adaptations that assure them a fair fight in the struggle for survival. The following sections describe a number of common adaptations that help insects survive adversity or adapt to their environment.
Migration
Day-to-day activities of insects often involve trivial movements associated with feeding, mating, or oviposition. These movements are “trivial” only in the sense that they are short-range, random in direction, and do not result in much dispersal of the population. At some time in their life cycle, however, many (perhaps most) of these insects will engage in a period of directional movement that carries them beyond the range of their local habitat. This long-distance movement, called migration, is a survival strategy with at least six potential advantages:
- escape from natural enemies
- find more favorable growing conditions
- reduce competition or relieve overcrowding
- locate new (or unoccupied) habitats
- disperse to alternate host plants
- reassort the gene pool to minimize inbreeding
Although most insects migrate by flying, a few species (chinch bugs, Mormon crickets, and armyworms, for example) travel on the ground. Migration by flight is often aided by prevailing winds. Once airborne, small insects may be lifted by thermal convection and carried hundreds of miles on frontal air masses. Even wingless individuals may be carried aloft by “ballooning” on silk threads or blowing off tall vegetation. Larger insects, like dragonflies and monarch butterflies, may control the direction of their migratory flights, but most smaller insects are carried wherever the wind blows them.
Migration is usually a distinct phase in the life cycle, almost always occurring before the onset of reproductive maturity. Migrants are innately programmed to move; they are not distracted by food or mates. Once the migratory urge is satisfied, the insect is generally in a physiological state to continue development or commence reproduction. Migration can be a very risky venture: in some species more than 90% of a population may die in transit. Despite such high mortality rates, the reproductive success of individuals who survive the trek apparently makes the gamble worthwhile for the species as a whole.
Diapause
The life cycle of many insect species may include a hormone-induced period of “dormancy” called diapause. Like hibernation in mammals, diapause is characterized by a reduction in oxygen consumption, metabolic rate, and physical activity. Feeding and growth are generally interrupted while the individual subsists on stored food reserves. Diapause typically occurs during the egg stage in some species, during a nymphal or larval instar in other species, or during the pupal stage in still other species. Even adults may enter a “reproductive diapause” which causes a significant delay in the onset of sexual maturity.
In temperate climates, many species enter diapause in the fall as an overwintering adaptation. Other species, however, have a summer diapause that helps them survive the dryness and/or heat. In either case, the onset of diapause is triggered by an environmental cue that precedes the adverse weather conditions (short daylengths in fall, for example). Diapause continues, even under apparently favorable conditions, until it is “broken” (terminated) by other environmental cues, such as long day lengths or exposure to a substantial period of low temperature.
Diapause is not always correlated with adverse environmental conditions. It can also regulate development within a population to ensure optimal timing of emergence or temporal synchrony with environmental resources. Female rabbit fleas, for example, have an obligate adult diapause that is broken only by feeding on the blood of a pregnant host rabbit. By the time the baby rabbits are old enough to be weaned, the flea’s offspring will be mature and ready to accompany the rabbits when they leave the nest. In this ecological relationship, diapause is an adaptation that keeps the flea population from exceeding the carrying capacity of its host.
Cold-hardiness
Since insects are poikilotherms (cold-blooded animals), their body temperature is usually similar to that of the air (or water) around them. Species that live in cold mountain streams (like mayfly naiads) or on the surface of ice and snow (like grylloblattids) are adapted for activity at low temperatures. Most other insects, however, slow down as the temperature falls.
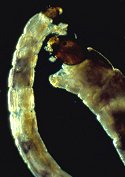
Belgica larvae
They reach a dormant state, called torpor or quiescence, when they get very cold. Physiologically, many insects prepare for winter weather by producing “antifreeze” compounds (such as glycerol, sorbitol, or trehalose) in their hemolymph and body tissues. High concentrations of these compounds can increase cold-tolerance by lowering the freezing point of body fluids and preventing the formation of ice crystals that would cause internal injury. In species that manage to survive in arctic and alpine environments, the overwintering stage may undergo extensive dehydration — any ice crystals that do form will be too small to cause cellular damage. During the long Antarctic winter, larvae of Belgica antarctica, a wingless midge, become literally frozen in place until the arrival of a spring thaw.
Unlike diapause (see above), a period of quiescence lasts only as long as the weather is cold. When temperatures rise, quiescent insects resume normal activity — at least until the next cold front arrives! This explains why there may be a great deal of insect activity on warm, sunny days in the middle of winter.
Parthenogenesis
Sexual reproduction involves the union of a female’s egg (1n) with a male’s sperm (1n) to form a diploid zygote (2n). Although sexual reproduction is the paradigm in most insects, there are many common species that are able to reproduce asexually (without mating), through a process known as parthenogenesis. In these species, females are able to produce viable offspring without a contribution of sperm from a male.
One type of parthenogenesis (called arrhenotoky) occurs in all members of the order Hymenoptera (ants, bees, and wasps) and also in a few species of thrips and scale insects. In these insects, all females are diploid (2n) and all males are haploid (1n). Mated females have voluntary control over the release of stored sperm so they can opt to lay either a fertilized egg (female) or an unfertilized egg (male). This adaptation, which allows a female to regulate the sex of her offspring, is undoubtedly an important factor in the evolution of colony structure for social ants, bees, and wasps.
Another type of parthenogenesis (called thelytoky) is found in many aphids, scale insects, some cockroaches and stick insects, and a few weevils. In these insects, females produce diploid (2n) eggs that develop directly into female offspring having exactly the same genetic make-up as their mother. Since each daughter subsequently has the ability to clone herself through parthenogenesis, this form of reproduction has the potential to produce a large number of offspring in a short period of time. Parthenogenesis is clearly an advantage for species that live in a stable environment and exploit an abundant food resource. The lack of genetic variability within the population, however, can be a disadvantage if (when) environmental conditions change. For this reason, many parthenogenetic species have evolved a seasonal cycle that includes at least one generation of sexual reproduction at the end of several generations of asexual reproduction.
Polymorphism
Just as each human has a unique physical appearance, there are also individual differences among the members of a single species in the insect world. Females are often larger than males, and one sex may have distinctive colors or markings to attract the opposite sex. But there are also many examples of species with two or more colors, shapes, or sizes where the differences are completely unrelated to gender characteristics. Each of these “versions” is called a morph, and therefore, species that exhibit this “split-personality” are said to be polymorphic.
In social insects, polymorphism is often associated with division of labor in the nest. Among ants, for example, large individuals with big mandibles usually serve as soldiers or foragers, while smaller individuals concentrate on care of the young or other housekeeping tasks. In honey bees, the workers have wax glands, stings, and pollen baskets that are not present in queens or drones. This type of specialization among individuals is an adaptation that gives social insects the ability to utilize their resources more efficiently.
In non-social species, polymorphism may be related to habitat diversity. England’s peppered moth, Biston betularia, is a well-known example of such a species. The light-colored morph of this moth is hard to find in the daytime when it rests against a background of lichens growing on the bark of trees. A dark-colored morph is easy to see against the lichen, but hard to spot against the dark background of bare bark. Depending on the background, the less-visible morph is the one most likely to survive bird predation. During the industrial revolution, the dark morph predominated because air pollution from London’s factories killed much of the lichen on trees in surrounding forests. Now that air pollution has been reduced, the lichen are able to survive and the moth’s light-colored morph is most abundant.
In Africa, the desert locust, Schistocerca gregaria, has two morphs that differ both in physical appearance and behavior. Under low population densities, these grasshoppers develop into adults that are largely green in color, have relatively short wings, and show little or no tendency to migrate. Under crowded conditions, however, these grasshoppers develop into brownish adults with longer wings. These individuals eventually form huge swarms containing millions of individuals that migrate over hundreds of miles. Crowding affects the balance of neurotransmitters, especially serotonin, and triggers a shift in development to the migratory form.
Different morphs may also be associated with different generations throughout the year. The seasonal cycle of many parthenogenetic aphids includes several generations of wingless (apterous) individuals followed by a generation of winged (alate) migrants. This alternation of generations provides a mechanism for dispersal from one habitat to another as environmental conditions and host plant quality change throughout the year. The rosy apple aphid, Anuraphis rosea, is typical of many such species. In early spring it reproduces asexually, completing several wingless generations on apple trees, its primary host. As the apple foliage matures and becomes less desirable, an alate generation develops (partheogenetically) and flies to narrow-leaved plantain, the secondary host. Several more wingless generations develop asexually on plantain until, in late summer or early fall, another alate generation develops and flies back to the primary host. This generation gives birth to a sexual generation (both males and females) that will mate and lay overwintering eggs on the apple trees.